Checking for non-preferred file/folder path names (may take a long time depending on the number of files/folders) ...
This resource contains some files/folders that have non-preferred characters in their name. Show non-conforming files/folders.
This resource contains content types with files that need to be updated to match with metadata changes. Show content type files that need updating.
Hydrologic and meteorological data from the Santa Fe River Sink-Rise flow system area, Florida, USA – May 2018-May 2021
Authors: |
|
|
---|---|---|
Owners: |
|
This resource does not have an owner who is an active HydroShare user. Contact CUAHSI (help@cuahsi.org) for information on this resource. |
Type: | Resource | |
Storage: | The size of this resource is 1.6 GB | |
Created: | Aug 02, 2023 at 4:06 p.m. | |
Last updated: | Aug 03, 2023 at 4:02 p.m. | |
Citation: | See how to cite this resource |
Sharing Status: | Public |
---|---|
Views: | 320 |
Downloads: | 9 |
+1 Votes: | Be the first one to this. |
Comments: | No comments (yet) |
Abstract
We conducted a three-year field study from May 2018 to May 2021 at the Santa Fe River Sink-Rise flow system in O’Leno and River Rise Preserve State Parks in north-central Florida, USA, that involved the collection of hydrologic, geophysical, and meteorological datasets and photos. We collected hydrologic data (water level, specific conductance, and temperature) at eight surface water bodies (River Sink, River Rise, and six intermediate karst windows), seven deep wells, and four shallow wells. The Santa Fe River sinks at River Sink, flows through conduits that are ~20 m in diameter and ~30 m below the surface, periodically reemerges at karst windows, and finally emerges at River Rise. We also collected meteorological data at a site in O’Leno State Park (wind speed, gust speed, wind direction, rain, atmospheric pressure, temperature, relative humidity, and dew point). All of the hydrologic and meteorological data are published here. Our instrument network also included 14 seismometers (12 short period (L-22) and two broadband (CMG 3T) seismometers), four borehole and two platform tiltmeters, and 149 nodal seismometers for a one-month campaign. Bilek (2018) provide the seismic data, and the tilt data will soon be available. Finally, we deployed five motion cameras near seismometer stations to identify sources of seismic signals at the surface. Photos are published here as a separate, but linked resource in this collection.
Subject Keywords
Coverage
Spatial
Temporal
Start Date: | |
---|---|
End Date: |
Content
readme.txt
HYDROLOGIC AND METEOROLOGICAL INSTRUMENTATION AND MOTION CAMERA DEPLOYMENT In May 2018, we deployed Solinst LTC Levelogger Edge M10 data loggers in seven surface waters (River Sink, River Rise, and five intermediate karst windows: Ogden Pond, Paraners Branch, Jug Lake, Hawg Sink, and Sweetwater Lake) to measure level, temperature, and conductivity and Solinst Levelogger Edge F30/M10 data loggers in four shallow wells to measure level and temperature (Tables 1 and 2). We also deployed a Solinst Barologger Edge F5/M1.5 data logger in the upper dry portion of Well 4A to measure atmospheric pressure and temperature, which allowed us to correct the water levels at all monitoring locations so that they only recorded changes from water levels. Ritorto et al. (2009) provide the well screen interval depths. Depths of sensors in the shallow wells ranged from ~5 to 10 m below the top of the well casing, and the top of the well casing is ~0.5 to 0.8 m above the ground level (Table 1). For the surface water locations, sensors were deployed in a PVC pipe that was fixed at an angle to a tree. The one exception was the sensor at River Sink, which was deployed in a PVC pipe that was vertical and fixed to a deck structure that allows a view of River Sink. Holes were drilled into the bottom part of the PVC pipes to serve as a well screen. In mid-July 2018, we installed Solinst LTC Levelogger Edge M30 data loggers to measure level, temperature, and conductivity in seven deep wells (Tables 1 and 2), four of which are adjacent to the shallow wells. Depths of these sensors ranged from ~25 to 28 m below the top of the well casing, and the top of the well casing is ~0.5 to 1 m above the ground level (Table 1). We deployed a Solinst LTC Levelogger Edge M30 data logger to measure level, temperature, and conductivity in Dog Leg (ephemeral surface water body) from Aug. 24-Sept. 20, 2019 (Tables 1 and 2). The hydrological sensors generally recorded data every two minutes up until the onset of the Covid-19 pandemic, after which the recording interval was generally reduced to every four minutes to limit trips to the field. Table 1 provides the dates and times of available data, output intervals, data logger characteristics (type and serial number), and sensor depths. During the experiment, sensors were replaced due to faulty batteries or sensor failure. Sensors were removed in May 2021. The full scale (FS) for the M10 and M30 sensors are 10 and 30 m, respectively. FS for the Barologger is air only (i.e., designed for use in air alone). At FS, the M10 level sensor has accuracy of 0.5 cm and resolution of 0.0006% FS, the M30 level sensor has accuracy of 1.5 cm and resolution of 0.0006% FS, and the Barologger has accuracy of 0.05 kPa and resolution of 0.002% FS. Temperature sensors for all models are accurate to 0.05 deg C and have a resolution of 0.003 deg C. Conductivity sensors for all models are accurate to the greater of 2% or 10 uS/cm from 50 to 5,000 uS/cm and have a resolution of 0.1 uS/cm. Conductivity readings are specific conductance normalized to 25 deg C. Our hydrologic dataset includes two master files and a hand measurement file for each hydrologic monitoring location. The first master file includes all of the raw data for each site, where we have compiled the monthly or bimonthly files into one master file while also including conversions to UTC time and some time corrections. The second master file includes corrected water level data to remove atmospheric pressure contributions and our best attempt to only include good data that we further processed and corrected. All of the data processing steps are described in more detail below. The hand measurement file includes the hand measurements that were measured and recorded when the data logger was serviced/downloaded. Our hand measurements included water level (m), conductivity (uS/cm), and temperature (deg C) that were measured with a Solinst 107 TLC Meter, with a temperature accuracy of 0.2 deg C, and the conductivity accuracy is the greater of 5% or 100 uS/cm. The conductivity readings are standardized to 25 deg C, with a conductance temperature coefficient of 2.0%/deg C. The hand measurement file also includes the percent battery remaining on the Levelogger at the time of servicing/download. The conductivity and temperature hand measurements up through March or April 2019 were likely made just below the water level surface and above the well screens (or drilled hole portions of the PVC pipes) and above where the Leveloggers would have been monitoring. Beginning with April or May 2019, the conductivity and temperature hand measurements were taken at approximately the same level as the Leveloggers in the screened portions of the wells or the drilled hole portions of the PVC pipes in the surface water bodies. We deployed a HOBO weather station on May 21, 2018 in the northern part of OLeno State Park (Table 1), and it collected data until May 19, 2021. The weather station measured and recorded wind speed, gust speed, wind direction, rain, atmospheric pressure, temperature, relative humidity, and dew point generally every minute, except for the first five days where the output interval was five minutes. The weather station started to malfunction on Jan. 10, 2019, which led us to send it back to the company for servicing before it started to collect data again on Feb. 26, 2019. We did not do anything to the data collected from our weather station, except for changing the wind speed and gust speed data to nan on June 14, 2018 15:49-15:50 when we did a spin test, which confirmed that the values changed. The weather station data are provided as four separate files that correspond to the calendar years of data collection with UTC time. The weather station consisted of a HOBO RX3003 3G Remote Monitoring Station that was charged with a 5 watt solar panel. The sensors included a barometric pressure sensor (maximum error of 5.0 mbar over -40 to 70 deg C and resolution of 0.1 mbar), a rain gauge (calibration accuracy of 1.0% at up to 20 mm/hr and resolution of 0.2 mm), a temperature/relative humidity sensor with a solar radiation shield (temperature accuracy of 0.21 deg C and resolution of 0.02 deg C at 25 deg C; relative humidity accuracy of 2.5-3.5% from 10% to 90% relative humidity and 5% below 10% and above 90% relative humidity and resolution of 0.1% relative humidity), and a wind speed and direction sensor set with a full cross arm kit (wind speed/gust speed accuracy of 1.1 m/s or 4% of reading, whichever is greater, resolution of 0.5 m/s, and starting threshold of 1.0 m/s; wind direction accuracy of 5 degrees, resolution of 1.4 degrees, and starting threshold of 1.0 m/s). We mounted all of the sensors on a 3 m tripod kit, and the data were uploaded remotely with a data plan. The motion cameras were deployed next to five of the seismometers (Table 1) in the Fall of 2018: Paraners Branch Broadband (PBB), Paraners Branch Center (PBC), River Rise North (RRN), River Rise South (RRS), and Sweetwater Lake West (SWW). The photos are published as a zipped file for each site in a separate resource in HydroShare and linked in this collection. The zipped files include all of the files that were saved from the SD card for each camera, which primarily consisted of photos in various folders. In addition, there are csv files that include the dates, times, and sources of motion that activated the cameras. There were times when the cameras were not working. Also, we were able to document pictures from site SWW while the pictures were temporarily available on a website, but we were unable to attain the photo files from the SD card on the camera when we removed it from the field. INITIAL QUALITY CONTROL AND PROCESSING We inspected graphs of the master files and monthly or bimonthly data files, and we removed data points that we deemed to be of poor measurement quality. This included the beginning of each monthly or bimonthly data file when the sensor reequilibrated after being placed back into the water and the last few measurements at the end of the files when the sensor was removed from the water for service/download. We deleted entire rows from the data files in this case. It took longer for the temperature and conductivity data to return to pre-disturbance conditions so we deleted additional temperature and conductivity data at the start of the data file. Because the water level data were of good quality, we did not deleted entire rows, but simply replaced poor temperature and conductivity data with nan during these times. In a few cases, we removed data points from the middle of the monthly or bimonthly files if there were clear outliers, possibly due to instrument malfunction. In other situations, entire or large portions of data files are not included in the processed data due to a dead battery, instrument malfunction, or unwanted physical tampering with the instrument by an unknown person or animal. Data points that were removed via these procedures may be determined by comparing the two master files for each site. Water level data needed post-processing to remove the atmospheric pressure contribution. These steps include: 1. Converting monthly atmospheric pressure data from the Barologger files from local to UTC time. Certain timestamps toward the end of the experiment were erroneous due to a computer clock malfunction (see the TIMING CORRECTIONS section below). 2. Compiling monthly Barologger atmospheric pressure data. 3. Converting monthly level, temperature, and conductivity data front the Levelogger files from local to UTC time. Certain timestamps toward the end of the experiment were erroneous due to a computer clock malfunction (see the TIMING CORRECTIONS section below). 4. Compiling monthly Levelogger level, temperature, and conductivity data. 5. Subtract the ratio of the atmospheric pressure (in kPa) to the specific weight of water (9.81 kN/m3) from the water level measurement (in m). Atmospheric pressure data points were linearly interpolated for times in which the Barologger data did not exactly match the time of hydrologic data points. We also corrected offsets in water level data between subsequent months (see the WATER LEVEL OFFSET ADJUSTMENT section below). TIMING CORRECTIONS Hydrologic data files downloaded during the final two downloads (in Feb 2021 and May 2021) have significant timing errors. These errors resulted from a clock malfunction in the field laptop used to download the data from the data loggers and to subsequently synchronize the data logger time with the laptop time. Specifically, the laptop failed to keep time when the screen was shut and the computer was also not connected to the internet prior to going to the field, which caused timing errors of up to several months behind the true time. Because the water level measurements contain information on the atmospheric pressure, we were able to cross-correlate these data with atmospheric pressure data recorded at the weather station or via Weather Underground (these data produced the same results as our weather station data at other times) for a period of time in which the weather station was down or not fully functioning (Jan. 10-Feb. 26, 2019). This process was completed for all service months and all stations to ensure accuracy of the results as well as to verify if the proper time zone correction was applied (i.e., standard or date time). Before completing cross-correlations on the final two obvious erroneous months, we adjusted those data to a best estimate time based on the hand measurement time entries on the service sheets as well as the end time of the previous months data file. For each service month, the cross-correlations were completed as follows: 1. Read in the weather station data. 2. Read in weather underground station KFLHIGHS13 (Weather Underground, 2021a) to be used for Jan. 10-Feb. 26, 2019 for stations that recorded an even minute. 3. Read in weather underground station KFLFORTW52 (Weather Underground, 2021b) to be used for Jan. 10-Feb. 26, 2019 for stations that recorded an odd minute. 4. Read in a monthly hydrologic data file. Timing was adjusted to the nearest minute in order to be consistent with the weather data. 5. Find where hydrologic and weather data intersect to make sure both datasets have the same data intervals. 6. Cut data into daily segments to stack cross-correlations. 7. Apply zero-phase 6th order butterworth bandpass filter with a low corner or 1 sample per 240 minutes and a high corner of 1 sample per (sample rate x 3) minutes, where the sample rate is either 1 sample every 2, 3, 4, or 5 minutes. 8. Cross-correlate and stack daily segments. 9. Add the max-time lag to the best estimate time, where the timing error is the max time-lag. WATER LEVEL OFFSET ADJUSTMENT Hydrologic sensors were connected to the caps of the well casings using a kevlar cord. During service runs, it was impossible to place the cap in the same position, the cords sometimes became knotted, and/or knots were removed from the cords. This led to several level offsets (up to several cm) in the data between service months. We identified and recorded the timing of these offsets, and we then adjusted them as follows: 1. Linear models were fit to the two days of data on either side of the data gap. In some cases, only models were fit to 12 hours of data on either side of the data gap, and the gaps this was applied to were determined qualitatively from the results. 2. The time of the middle of the gap was determined (that is, the time in the middle of the end of the first data file and the start of the second data file). 3. The data point for the time found in (2) was extrapolated using both models found in (1). 4. The difference between the extrapolated data points in (3) was calculated. 5. The calculated difference was added to all data points at later times of the gap. 6. The above steps were completed for all subsequent service months. 7. The adjusted levels were then adjusted based on an average offset between hand measurements, and hand measurements used in the calculation were determined qualitatively. 8. Stations that experienced one or more significantly large data gaps (days or more) were adjusted to hand measurements in chunks. These gaps were too large to be corrected using 1-6 above. REFERENCES Bilek, S. (2018). Geophysical characterization of karst aquifers using dynamic recharge events [Data set]. International Federation of Digital Seismograph Networks. https://doi.org/10.7914/SN/XS_2018. Ritorto, M., Screaton, E. J., Martin, J. B., & Moore, P. J. (2009). Relative importance and chemical effects of diffuse and focused recharge in an eogenetic karst aquifer: an example from the unconfined upper Floridan aquifer, USA. Hydrogeology Journal, 17(7), 1687-1698. Weather Underground (2021a). KFLHIGH13: Alachua County EM High Springs Fire WeatherSTEM Weather Data [Data set]. Weather Underground. https://www.wunderground.com/dashboard/pws/KFLHIGHS13. Weather Underground (2021b). KFLFORTW52: Fairview Estates Weather Data [Data set]. Weather Underground. https://www.wunderground.com/dashboard/pws/KFLFORTW52.
Related Resources
Title | Owners | Sharing Status | My Permission |
---|---|---|---|
Hydrologic and meteorological data and photos from the Santa Fe River Sink-Rise flow system area, Florida, USA – May 2018-May 2021 | Andrew Luhmann | Public & Shareable | Open Access |
Credits
Funding Agencies
This resource was created using funding from the following sources:
Agency Name | Award Title | Award Number |
---|---|---|
National Science Foundation | Collaborative Research: Geophysical characterization of a karst aquifer using dynamic recharge events | EAR 1850667 |
Contributors
People or Organizations that contributed technically, materially, financially, or provided general support for the creation of the resource's content but are not considered authors.
Name | Organization | Address | Phone | Author Identifiers |
---|---|---|---|---|
Joel Cuevas | ||||
Tatiana Summerall | ||||
Krista Van Der Velde | ||||
Riccardo Ansaldi | ||||
Alexander Janelle | ||||
Andrew Oberhelman | University of Florida | Florida, US | ||
Paul Bremner | ||||
Jared Ciarico | ||||
Lauren Madsen | ||||
Calvin Block | ||||
Personnel at O'Leno and River Rise Preserve State Parks | ||||
Florida Park Service |
How to Cite
This resource is shared under the Creative Commons Attribution CC BY.
http://creativecommons.org/licenses/by/4.0/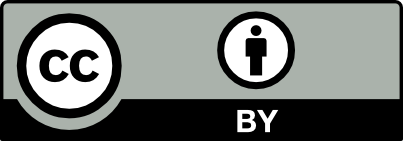
Comments
There are currently no comments
New Comment