Checking for non-preferred file/folder path names (may take a long time depending on the number of files/folders) ...
This resource contains some files/folders that have non-preferred characters in their name. Show non-conforming files/folders.
This resource contains content types with files that need to be updated to match with metadata changes. Show content type files that need updating.
Authors: |
|
|
---|---|---|
Owners: |
|
This resource does not have an owner who is an active HydroShare user. Contact CUAHSI (help@cuahsi.org) for information on this resource. |
Type: | Resource | |
Storage: | The size of this resource is 1.8 KB | |
Created: | Feb 07, 2023 at 6:55 p.m. (UTC) | |
Last updated: | Feb 07, 2023 at 6:55 p.m. (UTC) | |
Citation: | See how to cite this resource |
Sharing Status: | Public |
---|---|
Views: | 1237 |
Downloads: | 219 |
+1 Votes: | Be the first one to this. |
Comments: | No comments (yet) |
Abstract
Groundwater has been a part of the city of Santa Barbara's water-supply portfolio since the 1800s; however, since the 1960s, the majority of the city's water has come from local surface water, and the remainder has come from groundwater, State Water Project, recycled water, increased water conservation, and as needed, seawater desalination. Although groundwater from the Santa Barbara and Foothill groundwater basins only accounts for a small percentage of the long-term supply, it is an important source of supplemental water during times of surface-water shortages. During the late 1980s and early 1990s, production wells extracted additional groundwater to compensate for drought related water-delivery shortfalls from other sources; in response, water levels declined substantially in the Santa Barbara and Foothill groundwater basins (below sea level in the Santa Barbara groundwater basin). In coastal basins that have groundwater extraction near shore, seawater intrusion is often a problem. Seawater intrusion in the Santa Barbara groundwater basin is thought to be more limited than in other coastal basins because of an offshore fault that acts as a partial barrier to groundwater flow. During the late 1980s and early 1990s, seawater intrusion was observed in the Santa Barbara groundwater basin, as indicated by increased chloride concentrations at several monitoring wells that ranged from 200 ft to 1,300 ft from the ocean and as close as 2,900 ft to the nearest pumping well. This demonstrated that seawater can intrude into the Santa Barbara groundwater basin when groundwater levels fall below sea level near the coast. The city of Santa Barbara is interested in developing a better understanding of the sustainability of its groundwater supplies. In 2014, California adopted historic legislation to manage its groundwater: the Sustainable Groundwater Management Act (SGMA). The SGMA requires the development and implementation of Groundwater Sustainability Plans in 127 priority groundwater basins; although Santa Barbara was not a designated priority basin, the city is taking steps to achieve sustainability. Sustainability was defined in the SGMA in terms of avoiding undesirable results: significant and unreasonable groundwater-level declines, reduction in groundwater storage, seawater intrusion, water-quality degradation, land subsidence, and surface-water depletion. In this project, a cooperative study between the U.S. Geological Survey (USGS) and the city of Santa Barbara, sustainable yield is defined as the volume of groundwater that can be pumped from storage without causing water-level drawdowns and the associated increases in seawater intrusion (as indicated by increases in measured chloride concentrations) at selected wells. In order to estimate the sustainability of Santa Barbara's groundwater basins, a three-dimensional density-dependent groundwater-flow and solute-transport model (the Santa Barbara Flow and Transport Model, or SBFTM) was developed on the basis of an existing groundwater-flow model. To simulate seawater intrusion to the Santa Barbara Basin under various management strategies, the SBFTM uses the USGS code SEAWAT to simulate salinity transport and variable-density flow. The completed SBFTM was coupled with a management optimization tool, in this case a multi-objective evolutionary algorithm, to determine optimal pumping strategies that maximize the sustainable yield and at the same time satisfy user-defined drawdown and chloride-concentration constraints. As part of this study, a three-dimensional hydrogeologic framework model was developed to quantify the extent and hydrogeologic characteristics of the Santa Barbara and Foothill groundwater basins and to help define the discretization and hydraulic properties used in the SBFTM. The development of the hydrogeologic framework model required the collection and reconciliation of geologic and geophysical data from existing maps, reports, and databases, along with geologic and hydrologic data from recently drilled wells. These data were integrated into a three-dimensional hydrogeologic framework model that defines the stratigraphy and geometry of the aquifer zones and the major geologic structures in the basin. The hydrogeologic framework model also quantifies the variation in sediment grain size within each aquifer zone as the percentage of coarse-grained sediment. Previous studies indicated that there are two principal water-producing zones in the Santa Barbara groundwater basin, the upper and lower producing zones; an additional thin, productive zone was identified as part of this study. This middle producing zone is not as areally extensive as the upper and lower producing zones and only exists in the coastal part of Storage Unit I. These producing zones are bounded at depth by less productive shallow, middle, and deep zones. Two versions of the SBFTM were constructed: an initial-condition model and a modern transient model. The initial-condition model is a long-term transient model that simulates flow and solute-transport conditions during a period with limited anthropogenic influences preceeding the modern transient model. The simulation-transient model simulates flow and transport conditions from 1929 through 2013; however, because of data availability, the focus of the model calibration was 1972-2013. The SBFTM was calibrated to measured groundwater levels and drawdown, as well as measured chloride concentrations and change in concentrations, using a combination of automated and trial-and-error parameter-estimation techniques. A sensitivity analysis indicated that, in general, the SBFTM was most sensitive to recharge- and pumping-distribution parameters, specifically those controlling the amount of small-catchment recharge and the distribution of water extraction by hydrogeologic layer for production wells. The model was also sensitive to parameters controlling stream-recharge rates, horizontal and vertical hydraulic conductivity, and porosity. From 1929 to 1971, most of the water entering the area represented by the SBFTM was from creek and small-catchment recharge, and the majority of water leaving the SBFTM area was from pumping, discharge to creeks, and drains. In addition, about 37 percent of the total pumpage came from a net reduction in groundwater storage. From 1972 to 2013, the amount of water entering and leaving the SBFTM was fairly similar as that from 1929 to 1971, except the reduction in pumpage added about 17,000 acre-ft of water to storage. During this later period, there were also times of storage loss. For example, during July 1990, a month when approximately 705 acre-ft of groundwater was pumped in the study area, the pumpage was much greater than all sources of recharge combined, and about 382 acre-ft of water was removed from groundwater storage. Simulated hydraulic heads replicated the observed data to an acceptable matching of the measured water-level, flow direction, and vertical gradients. Simulated hydrographs for selected wells were in good agreement with the measured data, with an average residual of -2.7 ft and a standard deviation of 14.5 ft, indicating that the simulated heads, on average, underestimated the observed water levels. An examination of the model fit indicated that most of the discrepancies were lower simulated heads at wells proximal to production well sites. The simulated chloride concentrations reasonably matched the rising limbs of the measured breakthrough curves in terms of timing and magnitude; however, the simulation overestimated the chloride concentrations on the falling limbs. The overestimation of low chloride concentrations was attributed to the model overestimating the advance of the chloride front during periods of heavy pumping and underestimating the retreat of the chloride front during periods of low pumping. These simulation errors would result in a conservative response by local water managers to seawater intrusion. The SBFTM was used to develop a collection of predictive simulations optimized to produce pumping schedules that maximize yield, subject to a set of constraints and competing objectives. The simulations were grouped as scenarios that differed in their time horizon, initial conditions for groundwater levels and chloride concentrations, as well as precipitation, which was incorporated into the model through simulated recharge. Overall, five scenarios were developed in a multi-objective framework to obtain optimal pumping rates for all of the wells managed by the city, while minimizing excessive drawdown and seawater intrusion. For the current study, complexities in the simulation model and the optimization formulation required additional considerations. Incorporating the solute-transport equations to simulate chloride transport added a highly nonlinear process that is solved iteratively in each time step of the groundwater-flow model. These nonlinearities, coupled with the highly refined grid in the current model, creates challenges for many traditional optimization methods. Therefore, an optimization method was needed that could address nonlinear relationships as well as a very large problem size. Lastly, the optimization problem was reformulated to include multiple objectives without requiring convergence to a single solution. This approach, guided by the city's objectives, allowed the maximum extraction of information from the complex simulation. Borg, a multi-objective evolutionary algorithm, was chosen as the optimization algorithm for this study for several reasons: (1) it is very computationally efficient; (2) it can run in parallel; (3) it requires little user input; and (4) it can solve for multiple competing objectives. The first three points allow the algorithm to proceed toward the optimal solutions at the fastest possible rate. The fourth point is advantageous for large, complex optimization problems because it is difficult to formulate the optimization problem in a way that produces only one optimal solution. The problem formulation consisted of four competing objectives and a constraint set in accordance with the main concerns of the city. The objectives were maximizing total pumpage, minimizing seawater intrusion, minimizing total drawdown in production wells, and minimizing the maximum drawdown. The constraints were pump capacity, meeting drinking-water standards for chloride, maintaining a specified minimum flowrate to a groundwater treatment plant, and maintaining minimum water levels in pumping wells. The decision variables either were quarterly pumpage by well or total pumpage by basin. Five optimization scenarios were developed that allow the decision makers to evaluate a range of optimal solutions for a variety of water levels and chloride concentrations as well as potential future climatic conditions. Three scenarios (1, 2, and 5) were multi-objective optimization formulations that allowed for variations in management preferences and climatic conditions. The other two scenarios (3 and 4) were designed to examine the optimization results to answer specific questions. Scenario 1 described the best-case sustainable yield assuming a full basin (that is, high initial water levels) and typical climate conditions for 10 years. Scenario 2 also started with a full basin; however, this was followed by a 10-year drought. Scenario 3 determined if an empty basin (that is, low initial water levels) would recover to full conditions (1998 conditions) given climate assumptions and optimal pumping schedules from scenarios 1 and 2. Scenario 4 was designed to produce decision rules that can be used by water managers to help choose an optimal pumping schedule based on measured water-level or chloride data. Scenario 5 identified future pumping schedules based on short-term climate variations during a 2-year management horizon. The results from scenarios 1 and 2 described the differences in maximum pumpage in the basin under typical and dry long-term climate projections, respectively. The scenario 1 results indicated the maximum 10-year pumpage of the basin was about 31,300 acre-ft under typical conditions and controlling simulated seawater intrusion and drawdowns. For scenario 2, less recharge over the 10-year dry climate produced a maximum pumpage estimate of 30,000 acre-ft to control seawater intrusion and drawdowns. The larger pumpage for scenario 1 resulted in more seawater intrusion, but less total drawdown, compared to that of scenario 2. Results for scenarios 3 and 4 showed the basin's response to management actions combined with climate projections. Both scenarios used the optimal pumping schedules and the 10-year climates from scenarios 1 and 2. The scenario 3 results showed that under minimal pumping, the basin did not fully recover to 1998 water levels within 10 years under either climate scenario. The relatively larger recharge from the typical climate resulted in less drawdown at coastal monitoring wells after the 10-year recovery period than that from the dry climate. The location of the seawater intrusion front was not appreciably different between the scenarios, however. Scenario 4 used the optimal results from scenarios 1 and 2 to produce decision-rule curves that illustrated the pumpage for each basin, given measured levels of chloride concentration or drawdown. This allowed the use of additional measurements at monitoring wells to assess future management decisions on the basis of the sensitivity of observations of drawdown and seawater intrusion to various pumping rates. Scenario 5 allowed managers to investigate the effects of short-term climate variations on optimal pumping schedules. Three specific 2-year simulations were optimized: typical-to-dry (scenario 5A), dry-to-typical (scenario 5B), and dry-to-dry (scenario 5C). The most noteable result from scenario 5 was the overall reduction in optimal pumpage for most schedules in scenario 5C, when the climate is simulated as dry-to-dry. There are also many optimal pumping schedules that produced an overall increase in waterlevels over the two-year simulation period, regardless of climatic condition. Similar to scenario 2, the scenario 5C results represents conservative yield estimates under a minimal-precipitation climatic condition.
Subject Keywords
Coverage
Spatial
Content
Additional Metadata
Name | Value |
---|---|
DOI | 10.3133/sir20185059 |
Depth | 6.1 |
Scale | 11 - 101 km² |
Layers | > 20 layers |
Purpose | Groundwater resources;Groundwater contamination |
GroMoPo_ID | 83 |
IsVerified | True |
Model Code | MODFLOW;SEAWAT, MT3DMS |
Model Link | https://doi.org/10.3133/sir20185059 |
Model Time | 1929 - 2013 |
Model Year | 2018 |
Model Authors | S.R. Paulinski, T. Nishikawa, G. Cromwell, S.E. Boyce, Z. P. Stanko |
Model Country | United States |
Data Available | Report/paper only |
Developer Email | tnish@usgs.gov |
Dominant Geology | Unconsolidated sediments |
Developer Country | USA |
Publication Title | Santa Barbara and Foothill groundwater basins Geohydrology and optimal water resources management. Developed using density dependent solute transport and optimization models |
Original Developer | No |
Additional Information | |
Integration or Coupling | None of the above |
Evaluation or Calibration | Static water levels;Dynamic water levels;Groundwater chemistry |
Geologic Data Availability |
How to Cite
This resource is shared under the Creative Commons Attribution CC BY.
http://creativecommons.org/licenses/by/4.0/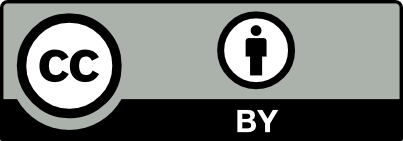
Comments
There are currently no comments
New Comment